An interdisciplinary group of UC Berkeley researchers are exploring the molecular mechanisms of friendship in prairie voles using fluorescent carbon nanotube sensors.
From parental care to mating systems, scientists have long relied on mouse models to study the critical biological pathways that underpin social relationships. But this model falls short when it comes to understanding what researchers call selective social preference—especially peer relationships that differ from more commonly studied reproductive relationships.
And it’s not just the animal model that falls short, but the tools available to scientists to study the complex molecular interactions that drive this behavior.
An interdisciplinary group of scientists from UC Berkeley are now beginning to tackle this technical gap unconventionally. Using single-walled carbon nanotubes—nanoparticles 1,000,000 times thinner than a piece of human hair—scientists can detect the chemical messengers that allow neurons in the brain to communicate with each other.
Because these tools don’t require genetic manipulation, researchers in the labs of Annaliese Beery and Markita Landry are now deploying them in prairie voles (Microtus ochrogaster), a species with the ability to develop selective peer relationships.
A preprint on the development of these nanosensors is published in BioRxiv, with a second manuscript on the behavioral work and in-vivo nanosensor data in preparation.
“These are same-sex peer relationships,” describes Lexi Black, a graduate student in the Beery lab. “In animals, we don’t usually call these relationships ‘friendships’ because it crosses some anthropomorphic lines, but we like to say that these selective relationships provide a good model for human friendships.”
Not unlike the way in which humans stick with the people they know at a party full of strangers, prairie voles form strong social preferences for familiar individuals or ‘partners’ that they have previously lived with. The researchers refer to the selective preference for peer partners as ‘peer bonds’, differentiating them from the term ‘pair bond,’ which is commonly used to describe selective mate relationships.
Black describes how they measure this social selectivity in their experimental design by quantifying “cuddling time” with strangers and peer partners in order to assess how various manipulations affect peer bond formation.
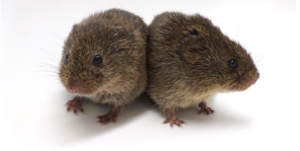
Neurochemical Underpinnings
The researchers aren’t just interested in the expression of these behaviors, but also their neurochemical underpinnings. Specifically, they’re interested in oxytocin, a chemical messenger long implicated in the formation and maintenance of selective relationships.
Neuropeptides like oxytocin act like messages sent between neurons, allowing for communication between cells in the brain. These messages dictate changes in the activity of neurons and modulate downstream responses. One way to study these pathways is to impair the receptor for oxytocin, which acts as the “inbox” for this chemical messenger on the receiving end of neural crosstalk.
The researchers accomplished this goal by using a genetically modified line of prairie voles containing a mutation in the oxytocin receptor gene that blocks expression of the respective receptors. Even though the brain is producing oxytocin, the message isn’t being received in the voles with the null oxytocin receptor.
By comparing wild-type, or un-modified, voles to their genetically modified counterparts, researchers can begin to disentangle the role of oxytocin signaling in peer social selectivity.
What the researchers find is a surprising breakdown in behavioral differences: When the voles are only allowed to cohabitate with their partners for 24 hours—a time sufficient for wild-type voles to form selective peer bonds—genetically modified voles do not form selective bonds over this interval. They cuddle with their partners less and show a reduced selective preference for their partner.
However, if the voles are allowed to cohabitate with partners for longer periods, up to a week, peer bond formation is recovered—both groups can form strong selective preferences for their peer partners. Essentially, selective pair bonding behavior is maintained when the genetically modified voles are allowed to bond for long periods of time, but, when these voles are only allowed to bond for short periods, they exhibit reduced selectivity in their bonding behavior compared to voles found in the wild. Pair bonding in peers is delayed, but not abolished. Given oxytocin’s long-purported role as a mainstay molecule for driving a host of social behaviors, it’s an unexpected finding that the oxytocin receptor null voles can still form these pair bonds if given additional cohabitation time. Not only this, but the result echoes other newly published work in vole social behavior.
Developing Nanoscale Tools
Delving into this question requires zooming in to the cellular level. But for a small molecule like oxytocin, this isn’t easy. That’s why these two labs, the Beery lab in the Department of Integrative Biology and the Landry lab in the Department of Chemical and Biomolecular Engineering, are working hand-in-hand to study social relationships from the behavioral level down to the subcellular scale.
“The firing of these neurons occurs on a sub-second scale,” describes Jaquesta Adams, a graduate student in the Landry lab. “And there’s usually a tradeoff involved in using traditional techniques to look at these neural communications. You can capture the spatial or temporal patterns in signaling, but not both.” That’s why the Landry lab has developed nanoparticle-based sensors for detection of these neurochemicals.
“You can think of these carbon nanotube sensors as tiny lamps we can put in the brain, and they only “light up” in the presence of our molecule of interest, in this case, oxytocin” explains Adams. “This fluorescent property is critical because it’s tissue transparent and reversible, so we can look deep into the brain in real-time.”
The key here is achieving molecular selectivity or ensuring that these nanoscale ‘lamps’ only fluoresce in the presence of the desired molecule. Researchers achieve this by wrapping sensors in a polymer, like DNA, that’s specific to oxytocin. The DNA acts like a blanket thrown over the lamp, and only the specific interaction with oxytocin can “pull it off,” revealing fluorescent signals. Now the two labs are working to deploy these sensors in prairie vole brain imaging to uncover oxytocin’s role in pair-bonding.
Nanosensors in the Brain
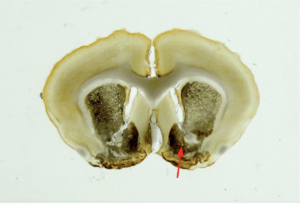
By applying these sensors to the wildtype and genetically modified voles, researchers can study whether or not compensatory changes in oxytocin signaling allow for maintenance of this social behavior—if the inbox is removed, can social behavior be recovered by sending out more (or different) messages? So far, nanosensor experiments reveal that this doesn’t seem to be the case for oxytocin. In fact, oxytocin release is actually lower in the genetically modified voles without intact receptors. So, if the voles aren’t releasing more oxytocin to compensate for their broken inboxes, how are they able to still form selective peer bonds?
It’s possible that the key to this maintained social behavior lies outside of purely molecular compensation, but in gene-by-environment interactions, or the compounding influence of environmental factors on genetically encoded behavior. Because the mutant voles are housed with wild-type animals with normal behavior, perhaps social behavior is learned and acquired over time, despite the genetic and neurological differences between the two groups.
“We’re beginning to build a better picture of the role of oxytocin in peer social behavior,” describes Black. “It seems as though oxytocin signaling isn’t necessary for selective relationships in the long-term, but rather it matters for the speed and efficiency of relationship formation.”
Oxytocin might not be the only key to unlocking social bonding, but it’s not totally out of the picture either—it potentially enhances the formation of social relationships in a way that allows them to form faster and stronger.
From an evolutionary perspective, social relationships are important for reproductive fitness and survival, so it makes sense that the persistence of these relationships doesn’t hinge on a single signaling pathway. Whether in voles or in humans, evolution builds in backups to ensure that these crucial social behaviors are maintained. These nanosensors aid in understanding these nuanced neural “backups.”
“We know that oxytocin has modulatory effects on dopamine and vice versa, and there’s some promiscuity in which neurochemicals bind to which receptors,” adds Natsumi Komatsu, a Landry lab postdoctoral fellow who contributed to the research. “Now we want to design our nanosensors to study these other neurochemicals in tandem with oxytocin.”
By leveraging both a novel tool and a unique model organism, researchers are finally beginning to shine a light on these invisible chemicals in the brain in order to disentangle the pathways that underlie social relationships. It’s a testament not only to the complexity of understanding these neural pathways, but to the innovative paths paved through interdisciplinary partnerships such as this one.
Sophia Tomatz is a graduate student in the Department of Plant and Microbial Biology at UC Berkeley and a member of the Landry Lab.