Algae are ubiquitous, diverse, and have developed a myriad of strategies to thrive in different environments. Graduate student Helen Liu discusses how scientists are using the diversity of algae to discover novel adaptations that enable survival in low-iron nutrition, a limiting trace element for photosynthetic organisms, in the hopes of increasing crop production in iron-starved regions.
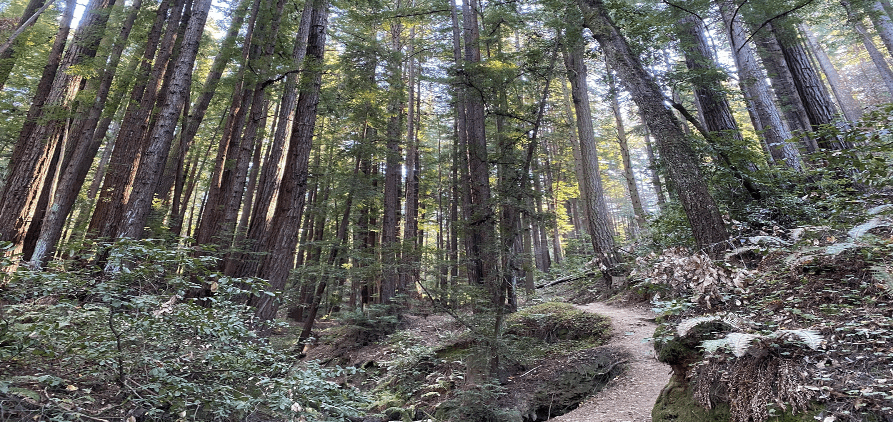
When you are walking through a redwood forest, what plants do you see? You will see the towering redwood trees that seal the skies with their over-reaching branches. Fuzzy-stemmed ferns and oxalis with their three, heart-shaped leaves might creep onto your path. Each plant species is different in their size, leaf shape, and growing habits. Even at the cellular level, plants differ in their genome size, the chemicals they produce, and the various shapes and sizes of their cells. What they all have common is a green organelle called the chloroplast. This organelle is where plants collect the energy of sunlight to convert carbon dioxide gas, the air we breathe out, into sugars that the plants can use for growth and reproduction in a process known as photosynthesis.
Photosynthesis is conducted by multiple proteins in a coordinated dance to transfer sunlight-excited electrons from one protein to another in order to produce the energy needed to convert gas into sugars. Within these proteins are single metal atoms, among them iron, that actually pass these excited electrons from one protein to another. Despite iron being a central component of photosynthesis and an abundant metal on Earth, iron is a limiting nutrient to plants in most environments. This is because iron’s chemical properties make it extremely difficult for plants to acquire in the presence of oxygen, especially in the case with rust. When photosynthetic organisms, like plants, no longer have enough iron for photosynthesis to continue, their leaves begin to yellow in a process called chlorosis as the pigments used to collect light in photosynthesis begins to break down. This reduces the productivity of plants; in crop plants, this translates to lower yields for farmers and less food for consumers.

Studying iron-nutrition in plants is not an easy task. Plants are composites of millions of cells that have differentiated to perform very specific functions, like forming a leaf, a root, a stem, or the beginnings of a flower. When a plant encounters a shortage of iron, how a root cell responds is very different to how a leaf cell responds. How then, can we easily and comprehensively study the plant response to iron-shortages without cell-function variation, such as at the single-cell level? There is a group of single-celled organisms that diverged from plants not too long ago which also have a plant-like chloroplast to perform photosynthesis: algae. Algae are a diverse group of photosynthetic organisms with some being very similar to plant cells in biochemistry, but also different to plants in other, key ways. Most importantly, we can study algae when they reproduce asexually, meaning the genetic information of the parent algae is exactly the same as the progeny, producing a large number of cells that are very similar to each other. Scientists can exploit this to study algae where every single cell is in the same stage of development and responds similarly to the environment, without the variation due to cell-function specialization. This makes algae an ideal candidate to understand plant iron-nutrition.

Fantastic algae, and how they survive
Algae are not the green monolith that we see in our uncleaned fish tanks, scum on freshwater lakes, or in the shaking laboratory flasks with green liquid. Under the microscope and at the genome-level, they are as diverse as the redwood trees and the ferns that we can observe with the naked eye. The many species of algae can be found in a myriad of environments such as the salt ponds of the San Francisco Bay Area (Dunaliella salina), embedded in sheets of ice in arctic glaciers (Chlamydomonas nivalis), or produce pigments that give flamingos and salmon their characteristic pink color (Chromochloris zofingiensis).
Iron is limiting in almost all of these environments, but each algae species respond to iron limitation in their own unique ways. Chlamydomonas reinhardtii, a soil-borne alga, has been used as a model organism to understand iron-nutrition biology. Chlamydomonas has the ability to use various sources of carbon, other than carbon dioxide, to produce food for itself, such as acetate, a component of vinegar. Using environmental carbon (acetate) for their growth and development has a lot of advantages for algae. Most-importantly, it allows the alga to be independent from light as the only source of energy. This flexibility dictates how this alga responds to low-iron nutrition depending on the food-source that is available.
When the algae are iron-starved and growing with acetate available, the algae will break down their photosynthetic proteins. The iron atoms previously employed in these broken down photosynthetic proteins are then utilized for other, more important processes that demand iron-dependent proteins, like extracting the energy from acetate through a process called respiration. In the reverse, where the algae are iron-starved but growing without acetate, and are thus completely reliant on the food produced from photosynthesis, the photosynthetic proteins are maintained as they are essential for its survival. In this way, the algae has two back-up plans for when iron suddenly becomes limiting, allowing them to wait out the shortage and survive until iron is available again.
Another interesting alga is the Dunaliella species, a not too distant relative of Chlamydomonas. Dunaliella can be found in high salinity regions, such as the salt ponds of the San Francisco Bay Area. They can be easily spotted through a plane window when one flies over the southern side of the San Francisco Bay Area from their characteristic orange pigments, carotenoids, that they accumulate in high light.
Dunaliella can only thrive on the sugar it produces from photosynthesis and cannot rely on environmental carbon sources like Chlamydomonas. Due to this, it has developed additional strategies to cope with iron-limitation. These include incorporating new proteins, like Tidi to the photosynthetic complex only during iron-limitation. Another specific iron-induced adaptation of Dunaliella is a protein that is commonly found in humans as an iron transport in the blood, transferrin. By expanding the diversity of algae that we study, we are bound to find novel and interesting ways algae have acclimated to constant low quantities of iron.
It’s greener on the other side
Algae, specifically green algae, share a common ancestor to plants. From this ancestor, one branch evolved into the plants that we see today while the other branch led to all the different algae we find underneath a microscope. The significance of this common ancestor is that many algal genes can also be utilized in plants, and vice versa. This means that the adaptations we find in algae can also be translated into plants, specifically into crop plants, using biotechnology techniques. Biotechnology allows us to introduce novel genes to generate whole new pathways in plants that cannot be introduced through traditional breeding. By teaching crop plants some of the tricks that algae use to survive and even flourish in iron-starved environments, we can extend the regions in which farmers can successfully grow crops, bringing the world closer to a more food-secure society. While studying and understanding the diversity of algae does not directly reap immediate benefits, the discoveries made in algae have the potential to be felt throughout global ecosystems, all of which depend in some way on plants to survive. Humans included.