Scientists are leveraging the modular nature of polyketide synthases (PKSs), or biologically-based, multi-domain enzymes, to design molecules in a way that could revolutionize everything from pharmaceuticals to biofuels.
In the world of chemistry, the smallest changes can have profound impacts. Altering even a single carbon atom in a molecule can completely change its function and how it interacts with its environment.
A group of scientists led by UC Berkeley professor Jay Keasling is pioneering a new approach to chemical synthesis using biology. Their focus? Polyketide synthases (PKSs) – modular proteins in which each domain is responsible for a different chemical reaction. Much like snapping together Lego pieces, researchers can rearrange PKS domains to create custom molecules.
One especially groundbreaking study from the lab recently published in Nature Catalysis and led by postdoctoral scholar Dr. Qingyun Dan focuses on producing diols and amino alcohols.
“These compounds have a wide range of applications, such as solvents, polymer building blocks, fragrances, cosmetic ingredients, and pharmaceutical intermediates,” explained Dan.
By harnessing the versatility of engineered PKSs, Dan and his colleagues developed a platform in Streptomyces albus capable of producing at least 17 distinct diols, amino alcohols, and 3-hydroxy acids – 13 of which had never been synthesized biologically before. This achievement not only opens the door to the creation of new-to-nature molecules but also offers potential environmental benefits by replacing products typically made with fossil fuels.
Biological building blocks
Minor structural changes in molecules often lead to major differences in their properties. For instance, slight alterations in a small molecule’s structure can turn a simple solvent into an insect repellent. Scientists have long sought sustainable ways to produce synthetic chemicals, and biosynthesis offers a promising alternative to traditional chemical synthesis.
This year, researchers in Keasling’s lab made significant progress in creating a flexible platform for synthesizing a wide variety of chemicals in microbial hosts. Typically, biological synthesis of new molecules requires extensive genetic engineering, with each product needing a specific pathway. Even slight modifications, like adding a single carbon atom, can require building an entirely new pathway from scratch.
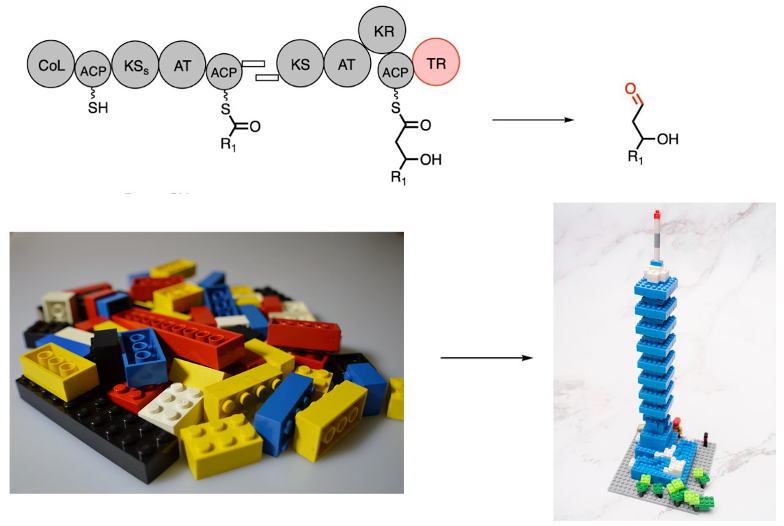
The Keasling lab is tackling this inefficiency by engineering a platform built around PKSs, which function like molecular assembly lines. Each domain in a PKS is responsible for a different reaction, allowing researchers to piece together these domains like building blocks and create entirely new molecules. This approach could greatly reduce the complexity and time required to design experimental products.
According to Dan, “Compared to traditional chemical synthesis, PKSs offer a distinct advantage in their ability to control and modify chemistry at specific positions within complex molecules, making their design space perhaps the largest of any biological synthesis pathway.” This capability allows engineers to access previously unattainable molecules.
The ability of PKSs to make products typically derived by petroleum-based processes makes them particularly valuable in industries looking for sustainable alternatives.
“Transitioning from petroleum-derived synthesis to PKS-driven microbial biosynthesis will be crucial in reducing greenhouse gas emissions and mitigating the environmental impact of industrial chemical production,” Dan emphasizes.
Novel termination chemistry
One particularly innovative aspect of this research lies in the choice of termination domains. The PKS carries the growing chemical product until the synthesis is complete, at which point the process ends with a terminating domain. While most natural modular PKSs terminate the synthesis with a thioesterase, which results in a carboxylic acid or triggers cyclization, Dan and his colleagues employed terminal thioreductases (TRs). TRs are associated with other mega-enzymes but were rarely found with modular PKSs.
Terminating the biosynthetic process with a TR generates an aldehyde, a functional group that offers new chemical possibilities not achievable with the more common thioesterease. By successfully incorporating a TR into the PKS platform in Streptomyces albus, Dan and his team were able to produce aldehyde derivatives with a broad range of potential applications. One example is 2-ethyl-1,3-hexanediol, an insect repellent and cosmetic ingredient that is traditionally challenging to synthesize.
Dr. Dan explained his excitement about this platform: “This work showed that it’s possible to fine-tune the PKS pathway with relative ease to control the final chemical product,” emphasizing how PKSs allow for precise control over minor modifications while also opening new avenues for biosynthesis.
Despite these advancements, some challenges remain. Dan points out two hurdles. “First, many PKSs tend to be relatively slow,” he admits. “To address this, we need efficient methods for rapidly screening large PKS libraries to identify the best candidates for producing specific target molecules.” Screening more PKS parts will help identify the best ones for making certain chemicals.
“The second issue is that PKS designs often fail to produce the desired products, and in many cases, the reasons for these failures are not well understood,” he adds. He suggests pairing experiments with advanced protein design tools, like AlphaFold 3, which was recently published by Google DeepMind. “By gaining a deeper understanding of PKS structure and catalysis, we can use this knowledge to inform rational design and improve the predictability and success of PKS-based biosynthetic designs.”
Dan’s paper tackles many of these challenges, pushing PKS engineering closer to industrial-scale applications. His research on diols, 3-hydroxy acids, and amino alcohols represents a crucial step towards making PKS-based platforms viable for large-scale chemical production.
As researchers continue to refine PKS engineering, this powerful platform could transform the chemical industry, offering new tools for making previously inaccessible products in more environmentally friendly ways.
Leah Keiser is a PhD candidate in Chemical and Biomolecular Engineering at UC Berkeley in Jay Keasling’s laboratory. Her research involves engineering microorganisms for sustainable chemical production.