A COVID-19 test is Dan Fletcher’s latest transformation of cell phones into mobile diagnostic tools.
Swab your nose, plunge the sample into a reaction chamber, insert the chamber into a cellphone-based device, then press “go.” In as little as 15 minutes, you’ll likely know whether you’re positive for COVID-19, with an unprecedented combination of speed and accuracy. If you’re positive, you’ll also learn how much viral load you’re carrying — valuable information about the severity and transmissibility of an infection. Additional research is underway to make the same test identify which variant of the ever-mutating coronavirus you’ve got.
So where can you go to get this test?
“The point is, you wouldn’t have to go anywhere,” says bioengineering professor Dan Fletcher, who developed the test in partnership with Berkeley’s Nobel Prize-winning biochemist Jennifer Doudna and UCSF professor of medicine Melanie Ott, director of the Gladstone Institute of Virology. “The whole goal is that it would be a point-of-care test — it would be right where people are.”
The lack of widely available testing has been a major roadblock to containing a virus that people can spread without showing, or even feeling, any symptoms. Having a quick, affordable and accurate test to place at hyper-local community locations — schools, airports, churches or even large gatherings like concerts or weddings — would be a critical new tool in controlling the pandemic, enabling the identification and isolation of carriers before they have a chance to spread the disease, which has killed more than 2.5 million people worldwide and caused economic and social upheaval.
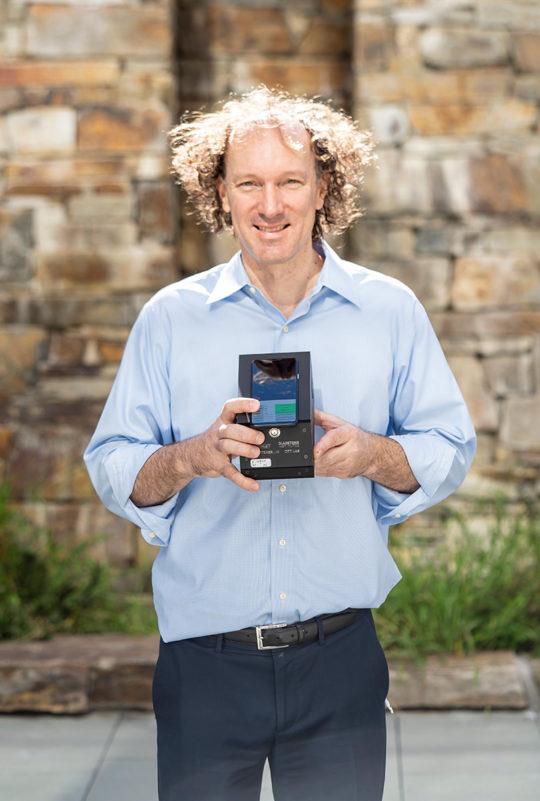
Several portable COVID-19 tests are in use or heading to market, but they are expensive, inconsistent and don’t quantify viral load. The smartphone’s advanced camera and processing abilities make the device uniquely suited to powering a mobile diagnostic lab. By adding some low-cost optical equipment, the Fletcher lab’s COVID device converts the smartphone camera into a microscope, and the phone’s powerful microprocessors support custom software that processes and analyzes results right on the phone instead of requiring a lab or computer to do so.
Standard-issue smartphone technology can geotag results, a potential aid in mapping the emergence of outbreaks and variants, and upload test data directly to a server. The ecosystem of apps and services promises even more connectivity in future iterations, Fletcher says, such as sending test data straight to an individual’s electronic medical record or a county public health office.
Retrofitting a smartphone is far less expensive than building a custom device with these same advanced capabilities because consumer tech companies keep upping their game, packing in more processing power and better features to entice consumers to upgrade. Fletcher is simply leveraging the mass-production of these mini supercomputers to build a sophisticated, portable medical device. “It’s really amazing — we’re just riding the wave of what consumers want,” he says.
The actual virus detection takes as few as five minutes. The reaction uses CRISPR, a technology that can locate specific genetic sequences inside cells, to send in specialized molecules to find the virus, as the project team documents in a study published in the journal Cell in January.
With support from the National Institutes of Health, Google.org and others, the team is preparing to scale up, making the components more manufacturing-friendly. A dedicated phone is the core of the device. “An app on that phone will run the whole system,” Fletcher says.
Flipping on the light switch
And what, exactly, is that system? When coronavirus RNA, which carries the genetic information the virus needs to replicate, is present in a sample, the camera, outfitted with optics for microscopy, captures images of fluorescence in the reaction chamber that it’s focused on. The result is quantitative — the more fluorescent the sample becomes across a series of time-lapsed images, the higher the viral load. No fluorescence, no virus. It sounds simple, but a lot of novel bioengineering is packed into that signaling mechanism.
In addition to co-developing CRISPR-Cas9 — the Nobel Prize-winning gene-editing technology that finds and cuts a specific sequence in the vast genetic material of DNA — the Doudna lab also demonstrated that another sequence-hunting enzyme, Cas13, has applications to virus detection. For their COVID test, the team sends in a Cas13 guide to look for coronavirus RNA in a sample. They prime the guide with a pair of molecules, configured by Doudna, that will fluoresce when the Cas13 enzyme snips them. The pair acts as a light switch set to the off position, programmed to flip on when Cas13 cuts the connection.
“We use that as a way of turning on a signal to say, ‘Yep, I’ve found what you told me to look for,’” Fletcher explains — in this case, coronavirus.
Key to the test’s speed and accuracy is a process called amplification — or rather, the lack of it. Standard COVID-19 laboratory tests convert viral RNA in a specimen into DNA, then copy it multiple times to make the DNA easier to find. However, amplifying a sample means the test doesn’t look at the actual virus information, just a transcribed, magnified version of it. That’s why current COVID testing can’t provide precise viral-load quantities, while the Fletcher lab’s direct-detection method — no transcription or amplification — can do so with great accuracy, and, Fletcher expects, it will significantly reduce the problem many tests have with false positives and negatives.
“Avoiding amplification avoids the problems with it — there’s just less noise there,” he says.
But skipping the amplification step requires a highly sensitive optical system to “see” the faint florescent light turning on. Fortunately, Sungmin Son, a postdoc in Fletcher’s lab since 2013 and co-lead author of the Cell study, is an expert in precision measurement tools.
Son, who earned his doctorate in mechanical engineering from MIT, sidelined his work on basic biological problems to focus on the COVID device, assembling the optics, a laser and a mobile phone camera that illuminates and detects even an extremely dim signal from a positive sample. With the pandemic’s urgency pressing on them, he and the team completed the task in about three months — lightning speed for research. The smartphone’s ready-made components were key to this speed, Son notes, allowing prototyping without having to assemble a device with its own camera, circuits and processors.
María Díaz de León Derby, a doctoral candidate in the UC Berkeley-UCSF joint bioengineering program and co-lead author with Son and the Ott lab’s Parinaz Fozouni, notes that while a few labs have used Cas13 to detect viral RNA, doing so without amplification is new. “We showed that we can get to a very sensitive level of detection without pre-amplification,” she says.
She also felt intense time pressure, but notes that the project’s payoff will extend well beyond COVID-19. “We now have this detection system we can use to find other diseases,” she says. “One of the coolest things about our technique is that to switch to a different disease, you really just have to change the RNA sequence you’re looking for.”
An ethos of collaboration
The quick progress also reflects the Fletcher lab’s culture of collaboration. In a typical hyperspecialized academic environment, Son says, he’d simply publish his method, then wait to see how it was applied. In Fletcher’s lab, he says, “You can actually see the applications of your research within your own lab.” And, he adds, it goes both ways. “The questions people are asking become my inspiration for developing a new technique.”
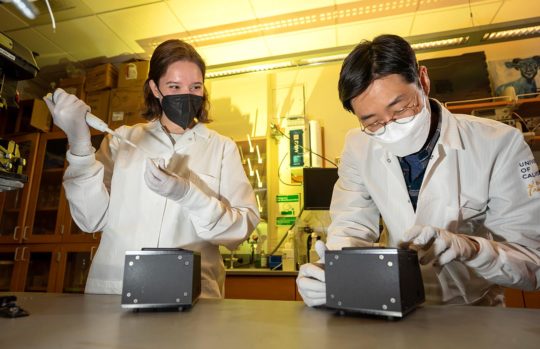
Other specialties in the lab include nanotechnology, biomedical engineering, biophysics and chemistry. “As we try to answer basic questions and solve real problems, we need perspectives from different fields and life experiences,” Fletcher says. He also values enthusiasm. “Much of the material — the facts, the systems — can be learned,” he says. “But it’s very hard to teach excitement.”
Fletcher, who once imagined a career pursuing basic questions from the inside of a microscope room, says his work with the Ott and Doudna labs has convinced him that collaboration is central to solving big problems.
And, while he initially didn’t set out to focus on public health, the real-world applications of his work have been exciting and also have brought intellectual rewards. “I’m finding that addressing practical problems can raise fundamental questions,” he says. “How do we rapidly detect the presence of a virus? How do we identify the presence of other pathogens? They involve very basic questions about how enzymes work and how infectious diseases and their hosts try to outsmart each other. I find those questions fascinating.”
The power of diagnosis
Fletcher, Ott and Doudna were able to get the COVID test moving as early as February 2020 because they already had been collaborating on an HIV test with the same components — a Cas13-based fluorescent light switch that avoids amplification.
The HIV-detection work was itself an outgrowth of Fletcher’s dozen-plus years of cell-phone-based microscopy at UC Berkeley’s Blum Center for Developing Economies. That work started, as many great discoveries do, accidentally — getting a cellphone camera to take microscopic images was a last-minute assignment for his Optics and Microscopy class. Working with his students on the project, Fletcher realized he was onto something, and the work that would become the CellScope quickly developed into one of the major thrusts of his lab, together with basic biophysical research on pathogens and the immune system.
One major CellScope application detects, in minutes, the presence of the Loa loa worm in a simple finger-prick blood sample. The worm can cause dangerous, even deadly side effects to an otherwise safe treatment for river blindness, a devastating parasitic infection that is a leading cause of preventable blindness in the world and affects mainly developing countries in sub-Saharan Africa. In a 2015 pilot in Cameroon, local health workers used Fletcher’s device, named the LoaScope, to clear more than 15,000 patients for immediate — and safe — river blindness treatment. The Gates Foundation is supporting a scale-up of the device, and the Fletcher lab is piloting the same the system to detect schistosomiasis, another parasite-transmitted tropical disease.
While the lab also is exploring CellScope modifications to detect malaria and tuberculosis, Fletcher’s current priority is so-called neglected tropical diseases.
“Malaria and tuberculosis get more attention because they’re killers,” he says. “For neglected tropical diseases, the death toll is not as significant, but the impact on quality of life is tremendous.” People don’t generally die directly from river blindness, he says, “But if you’re blind in a region where there’s zero support for anyone with disabilities, and if you’re a burden on your family and you can’t work, these diseases reinforce poverty.”
And because such diseases are treatable, there’s potential for enormous progress. “Treatments can be provided if we know which patients need what,” he says. “That’s why the diagnostics are so important.”
Decentralizing healthcare
Fletcher envisions a world where affordable, mobile diagnostic tools like his are ubiquitous and, eventually, even work on our personal phones. Such tools can help bring healthcare equity to perpetually underserved communities, from the 46 million Americans who live rurally to the under-resourced urban communities that have seen less care and more illness and death from COVID-19.
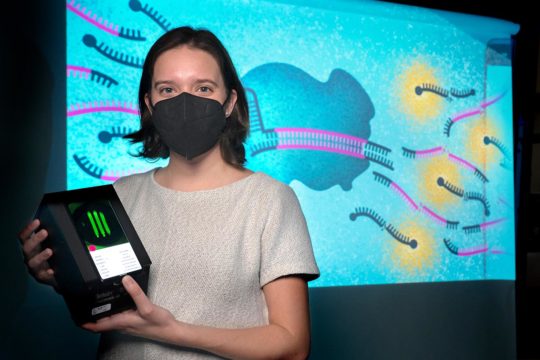
“I hope the silver lining of the pandemic is that we rethink the centralized clinical laboratory approach to taking a measurement and figuring out if someone is infected with something,” Fletcher says.
In addition to diagnostic power, more far-reaching and efficient data collection could lead to research insights about how immune systems behave based on who we are, and where and how we live, he says — information that could help mitigate social disparities in health and efficiently document disease outbreaks.
Arguably, such technology-driven decentralization has already started, with the coronavirus’s extreme transmissibility increasing the demand for telemedicine. Our cell phones have enabled decentralization in other fields — we flash e-tickets instead of lining up at airport counters and venue box offices; we deposit checks from home. Why not employ mobile phones for healthcare?
And, while tools like the LoaScope can help to disrupt entrenched cycles of poverty for some of the poorest and most remote populations in the world, “the pandemic has revealed many of the problems that plague all of us,” Fletcher says, such as testing and treatment limitations. “We started working in developing countries because that’s where the need seemed most urgent,” he says, “but it’s now become clear that the need is everywhere.”
This story was reposted from Berkeley Engineering. Read more here.